Chapter 14: Observations
Skip chapter table of contents and go to main content


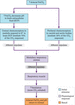
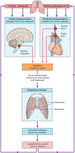
Anatomy and physiology
The organs and structures of the respiratory system can be split into two zones: the conducting zone and the respiratory zone (Marieb and Hoehn [110]). The conducting zone consists of a series of interconnecting passageways, both outside and within the lungs, through which air passes to get to the area of gaseous exchange, such as the nasal cavity, trachea and bronchi (Marieb and Keller [111]). The function of this area is to provide conduits through which air can pass, and it can also filter, cleanse, warm and humidify the air while conducting it to the lungs (Tortora and Derrickson [199]). The respiratory zone consists of the bronchioles, alveolar ducts and alveoli, which are the main sites where gaseous exchange occurs (Marieb and Hoehn [110]).
A variety of efficient inter‐relationships exist between the different body systems in order for effective respiration to occur; these include the cardiovascular system, nervous system and musculoskeletal system (Patton [155]). The respiratory muscles (the diaphragm and intercostal muscles) promote ventilation by causing volume and pressure changes within the respiratory system (Marieb and Hoehn [110]).
The mechanism of breathing
The key mechanism of pulmonary ventilation is that changes of volume within the respiratory system lead to changes in pressure, and these in turn lead to a flow of gases to equalize the pressure (Marieb and Hoehn [110]). The relationship between the pressure and volume of a gas is called Boyle's law, whereby at a constant temperature the pressure of a gas varies inversely with its volume; in a larger container, the pressure of a gas is less than in a smaller one (Marieb and Hoehn [110]). As air will flow from an area of high pressure to an area of lower pressure, air moves into the lungs when the air pressure inside the lungs is lower than the air pressure in the atmosphere, and out of the lungs when the air pressure outside the lungs is greater than the air pressure in the atmosphere (Tortora and Derrickson [199]).
Inspiration
For inspiration to occur, the pressure in the alveoli must be lower than the air pressure in the atmosphere; this is achieved by increasing the size (volume) of the lungs through the contraction of the muscles of inhalation – the diaphragm and the intercostal muscles (Marieb and Keller [111]). The diaphragm flattens and descends and the intercostal muscles lift the ribcage and sternum, causing the ribs to broaden outwards and increasing the diameter of the thoracic cavity, both from side to side and from front to back (Patton [155]). During normal inspiration, the pressure between the two pleural layers in the pleural cavity (intrapleural pressure) is always just lower than atmospheric pressure (756 mmHg at an atmospheric pressure of 760 mmHg) (Marieb and Hoehn [110]). As the volume of the lungs and pleural cavity increases, the intrapleural pressure drops to 754 mmHg (Marieb and Hoehn [110]). At the same time, the pressure within the lungs (alveolar or intrapulmonary pressure) drops from 760 mmHg to 758 mmHg, and so a pressure difference between the atmosphere and the alveoli is established and air flows into the lungs (Tortora and Derrickson [199]). The relationship between these pressures can be seen in Figure 14.27.

Figure 14.27 Pressure changes in pulmonary ventilation. Source: Reproduced from Tortora and Derrickson ([199]) with permission of John Wiley & Sons.
Expiration
Expiration also occurs due to a pressure gradient but in the opposite direction, such that the pressure within the lungs is greater than the pressure of the atmosphere (Patton [155]). In normal gentle breathing, the process of expiration is largely passive, not involving any muscular contractions but resulting from elastic recoil of the chest wall and lungs (Tortora and Derrickson [199]).
Expiration begins when the inspiratory muscles relax, causing the diaphragm to move superiorly and the ribs to depress, which decreases the diameter of the thoracic cavity and returns it to its normal size (Marieb and Keller [111]). This results in a decrease in lung volume and compression of the alveoli, causing an increase in alveolar (intrapulmonary) pressure to 762 mmHg (Tortora and Derrickson [199]). This forces gases to flow out from the area of higher pressure in the lungs to the area of lower pressure in the atmosphere (Tortora and Derrickson [199]). A summary of the events that occur during inspiration and expiration can be seen in Figure 14.28.

Figure 14.28 Summary of events of inhalation and exhalation. Source: Reproduced from Peate and Wild ([157]) with permission of John Wiley & Sons.
The accessory muscles
The accessory muscles of inspiration further increase the volume of the thoracic cavity and, therefore, increase the volume of breathing. These muscles include the sternocleidomastoid muscles, which elevate the sternum, and the scalene muscles and the pectoralis minor muscles, which elevate the first to the fifth ribs (Tortora and Derrickson [199]). These muscles may be used during exercise or in situations where the individual is in respiratory distress (Marieb and Hoehn [110]).
The accessory muscles of expiration are used only during forceful breathing, such as when playing a wind instrument or during exercise (Tortora and Derrickson [199]). Contraction of the abdominal wall muscles, primarily the oblique and transversus muscles, increases intra‐abdominal pressure, forcing the abdominal organs upwards against the diaphragm, and also depresses the ribcage (Waugh and Grant [207]). The internal intercostal muscles also depress the ribcage and decrease thoracic volume, forcing air out of the lungs (Marieb and Hoehn [110]).
Control of respiration
Oxygen consumption increases exponentially during periods of strenuous exercise; several mechanisms help to match respiratory effort to the metabolic demand of the cells (Tortora and Derrickson [199]). Higher brain centres, chemoreceptors and other reflexes modify the basic respiratory rhythms generated in the brainstem (Marieb and Keller [111]). Control of respiration primarily involves neurons in the medulla and pons (Marieb and Hoehn [110]).
The respiratory centres
Within the medulla are two clusters of neurones that are critically important in the co‐ordination of the respiratory system: the dorsal respiratory group (DRG) and the ventral respiratory group (VRG) (Marieb and Hoehn [110]).
The DRG contains neurones that generate impulses; they stimulate the diaphragm and the external intercostal muscles to contract, via the phrenic and intercostal nerves, and contraction of these muscles leads to inspiration. Generation of the impulses is then paused, allowing the diaphragm and intercostal muscles to relax, leading to passive expiration (Marieb and Hoehn [110]). The VRG contains neurones that act as ‘pacemakers’ and control the rhythm of breathing (Marieb and Hoehn [110]). It is also from the VRG that active or forceful breathing is controlled (Tortora and Derrickson [199]).
The DRG co‐ordinates input from the peripheral baroreceptors (or stretch receptors) and chemoreceptors and relays this to the VRG to alter respiratory rate as required (Marieb and Hoehn [110]). Baroreceptors monitor the stretch of the bronchi and bronchioles during overinflation of the lungs (Tortora and Derrickson [199]). Chemoreceptors monitor the arterial blood for changes in the partial pressure of carbon dioxide (PaCO2) and pH, but also, to a lesser extent, the partial pressure of oxygen (PaO2) (Patton [155]). For more information on the chemical factors that affect respiration, see Chapter c12: Respiratory care, CPR and blood transfusion.
Additionally, pontine respiratory centres relay impulses to the VRG to modify breathing rhythms so that there is a smooth transition from inspiration to expiration and so that breathing can be modified to allow for speech, exercise and sleep (Marieb and Hoehn [110]).
Carbon dioxide
Although oxygen is essential for every cell in the body, the body's need to rid itself of carbon dioxide is the most influential stimulus to respiration in a healthy person (Marieb and Hoehn [110]). Arterial PaCO2 is closely monitored and acceptable levels maintained by a sensitive homeostatic mechanism mediated mainly by the effect that increased carbon dioxide levels have on the central chemoreceptors of the brainstem (Tortora and Derrickson [199]). Carbon dioxide passes easily from the blood into the cerebrospinal fluid, where it forms carbonic acid, releasing hydrogen ions (H+) (Marieb and Keller [111]). This increase of H+ causes the pH to drop, stimulating the central chemoreceptors in the brainstem to increase the rate and depth of breathing and so increase the amount of carbon dioxide exhaled (Marieb and Hoehn [110]). Similarly, should a metabolic acidosis or acidaemia (low pH) exist (such as a build‐up of lactic acid), a change in respiration will ensue in an attempt to compensate for this (Marieb and Hoehn [110]). See Figure 14.29 for negative feedback mechanisms by which changes in PaCO2 and blood pH regulate ventilation. Abnormally low PaCO2 levels cause inhibition of respiration, with breathing becoming slow and shallow, and periods of apnoea may occur until arterial PaCO2 rises again and stimulates respiration (Marieb and Hoehn [110]).
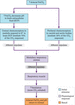
Figure 14.29 Negative feedback mechanisms by which changes in PaCO2 and blood pH regulate ventilation. Source: Marieb and Hoehn ([109]).
Oxygen
The peripheral chemoreceptors, found in the aortic arch and carotid arteries, are responsible for sensing the arterial PaO2 (Marieb and Hoehn [109]). Normally, decreasing levels of oxygen only affect respiratory rate by causing the peripheral chemoreceptors to have an increased sensitivity to carbon dioxide (Marieb and Hoehn [110]). A normal arterial PaO2, regardless of age, should be greater than 10.6 kPa (80 mmHg); a significant drop will cause the central receptors to become suppressed and at the same time the peripheral receptors will stimulate the respiratory centres to increase ventilation even if PaCO2 is normal (Marieb and Hoehn [110]).
Other ways in which respiration is controlled
There are a number of receptors in the lungs that respond to a variety of irritants (Marieb and Keller [111]). Accumulated mucus or inhaled debris stimulates receptors in the bronchioles that cause reflex constriction of those air passages (Tortora and Derrickson [199]). When these receptors are stimulated in the bronchi or trachea, a cough is initiated, whereas when they are stimulated in the nasal cavity, a sneeze is triggered (Marieb and Hoehn [110]). These irritant receptors have a protective mechanism to prevent obstruction or aspiration of food or liquids (Patton [155]). There are also stretch receptors present in the conducting passages and the visceral pleura that are stimulated when the lungs inflate; these then signal the respiratory centres to end inspiration (Marieb and Hoehn [110]). These receptors are thought to act more as a protective response to prevent excessive stretching of the lungs than as a normal regulatory mechanism (Marieb and Hoehn [110]).
Respiration can also be altered in the higher cortical centres in response to factors such as strong emotions, pain and alteration of temperature (Tortora and Derrickson [199]). The cerebral motor cortex yields a degree of voluntary control over breathing; however, this can be overridden by the other mechanisms (Patton [155]).
Some of the mechanisms through which breathing is controlled are summarized in Figure 14.30.
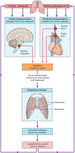
Figure 14.30 Factors that influence rate and depth of breathing. Source: Marieb and Hoehn ([109]).